In the ever-evolving world of manufacturing, technology continues to reshape how products are designed, prototyped, and produced. One such revolutionary advancement is Selective Laser Sintering (SLS) 3D printing, a technology that’s not just a buzzword but a game-changer in the manufacturing arena. But what exactly is SLS 3D printing, and why is it garnering so much attention?
SLS 3D printing is a cutting-edge additive manufacturing process that uses a laser to sinter powdered material, binding it together to create a solid structure. Unlike traditional manufacturing methods, which often involve cutting away material, SLS builds objects layer by layer, allowing for unprecedented design flexibility and material efficiency.
The roots of SLS technology stretch back to the mid-1980s, when Dr. Carl Deckard, a graduate student at the University of Texas, pioneered the concept as part of his thesis. Since then, it has evolved significantly, becoming a cornerstone technology for industries ranging from aerospace to medical devices.
In today’s competitive landscape, understanding the advantages of SLS in modern manufacturing is crucial. Not only does it offer remarkable design freedom and material versatility, but it also supports the production of functional prototypes and end-use parts with reduced waste and cost efficiency. As we delve deeper into the intricacies of SLS 3D printing through this blog, you’ll discover why it’s a vital tool for businesses aiming to innovate and scale in the digital age.
An In-Depth Overview of SLS 3D Printing
As the manufacturing landscape continues to evolve, Selective Laser Sintering (SLS) remains at the forefront of innovation, offering unparalleled possibilities for designers and engineers alike. But how exactly does this fascinating technology work, and how does it compare to other 3D printing methods?
How Does SLS 3D Printing Work?
At its core, SLS 3D printing is an additive manufacturing process that uses a high-powered laser to fuse small particles of polymer powder into a solid structure. Here’s a step-by-step breakdown of the process:
- Design Preparation: The process begins with a 3D CAD model that is digitally sliced into thin layers.
- Material Layering: A thin layer of powder is spread evenly across the build platform.
- Laser Sintering: A laser selectively fuses the powder according to the digital design. The laser traces the cross-section of the object, sintering the particles together.
- Layer Repetition: The platform lowers slightly, and a new layer of powder is applied. The laser continues to sinter each successive layer until the part is complete.
- Cooling and Post-Processing: Once the build is complete, the entire bed cools down before excess powder is removed and parts are retrieved.
Key Advantages:
- No Need for Support Structures: The surrounding powder provides natural support, allowing for complex geometries without additional supports.
- High Precision and Detail: The laser allows for high precision, enabling detailed and intricate designs.
Materials Used in SLS
SLS technology is compatible with a variety of materials, offering versatility in applications. Some commonly used materials include:
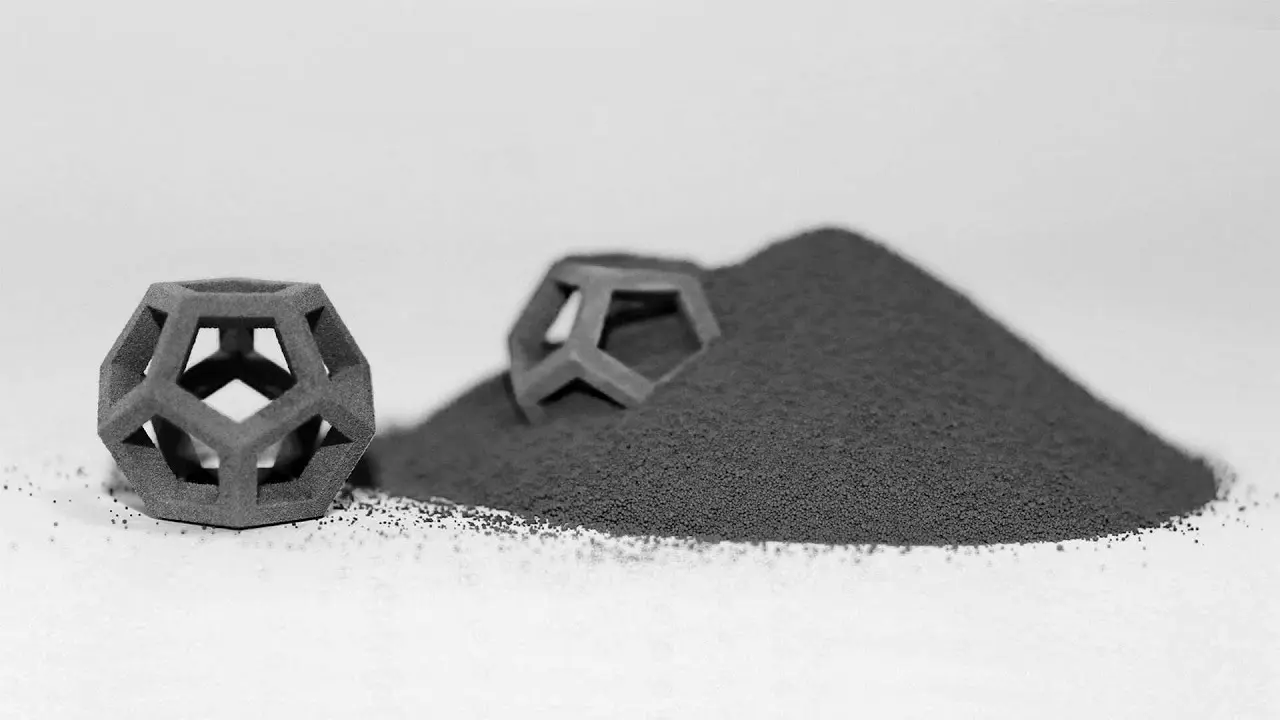
- Nylon (Polyamide): Known for its strength and flexibility, nylon is one of the most popular materials in SLS.
- Glass-Filled Nylon: Offers increased rigidity and thermal resistance.
- TPU (Thermoplastic Polyurethane): Provides rubber-like elasticity, ideal for flexible parts.
- Polystyrene: Often used for lightweight applications.
Material | Properties | Applications |
Nylon | Strong, flexible, durable | Functional prototypes, end-use parts |
Glass-Filled Nylon | Rigid, heat-resistant | Automotive components, housings |
TPU | Elastic, impact-resistant | Seals, gaskets, shock absorbers |
Polystyrene | Lightweight, easily finished | Models, lightweight structures |
Comparison with Other 3D Printing Technologies
While SLS is a powerful tool, it’s important to understand how it stacks up against other 3D printing methods like SLA (Stereolithography) and FDM (Fused Deposition Modeling).
- SLA (Stereolithography)
- Process: Uses a UV laser to cure liquid resin into solid layers.
- Best For: High-resolution, detailed parts with smooth surface finishes.
- FDM (Fused Deposition Modeling)
- Process: Melts and extrudes thermoplastic filaments to build layers.
- Best For: Simple prototypes, cost-effective production.
Technology | Precision | Material Variety | Speed | Surface Finish |
SLS | High | Moderate | Moderate | Rough |
SLA | Very High | Limited | Slow | Very Smooth |
FDM | Moderate | High | Fast | Moderate |
Why Choose SLS?
- Complex Geometries: SLS excels in creating parts with intricate details and complex geometries that might be difficult to achieve with FDM or SLA.
- Durability: Offers strong and durable parts suitable for a wide range of applications.
- Scalability: Ideal for producing both prototypes and end-use parts, making it a versatile option for various production needs.
In conclusion, SLS 3D printing is a transformative technology that offers distinct advantages in design flexibility, material usage, and production capabilities. Whether you’re in aerospace, automotive, or consumer goods, SLS opens up a world of possibilities for innovation and efficiency.
Unlocking Design Freedom with SLS 3D Printing
One of the most compelling advantages of Selective Laser Sintering (SLS) 3D printing is the design freedom it offers. Unlike traditional manufacturing methods that often impose limitations on design complexity, SLS opens up a world of possibilities for engineers and designers. But what does design freedom really mean in the context of SLS, and how does it revolutionize the way we create and innovate?
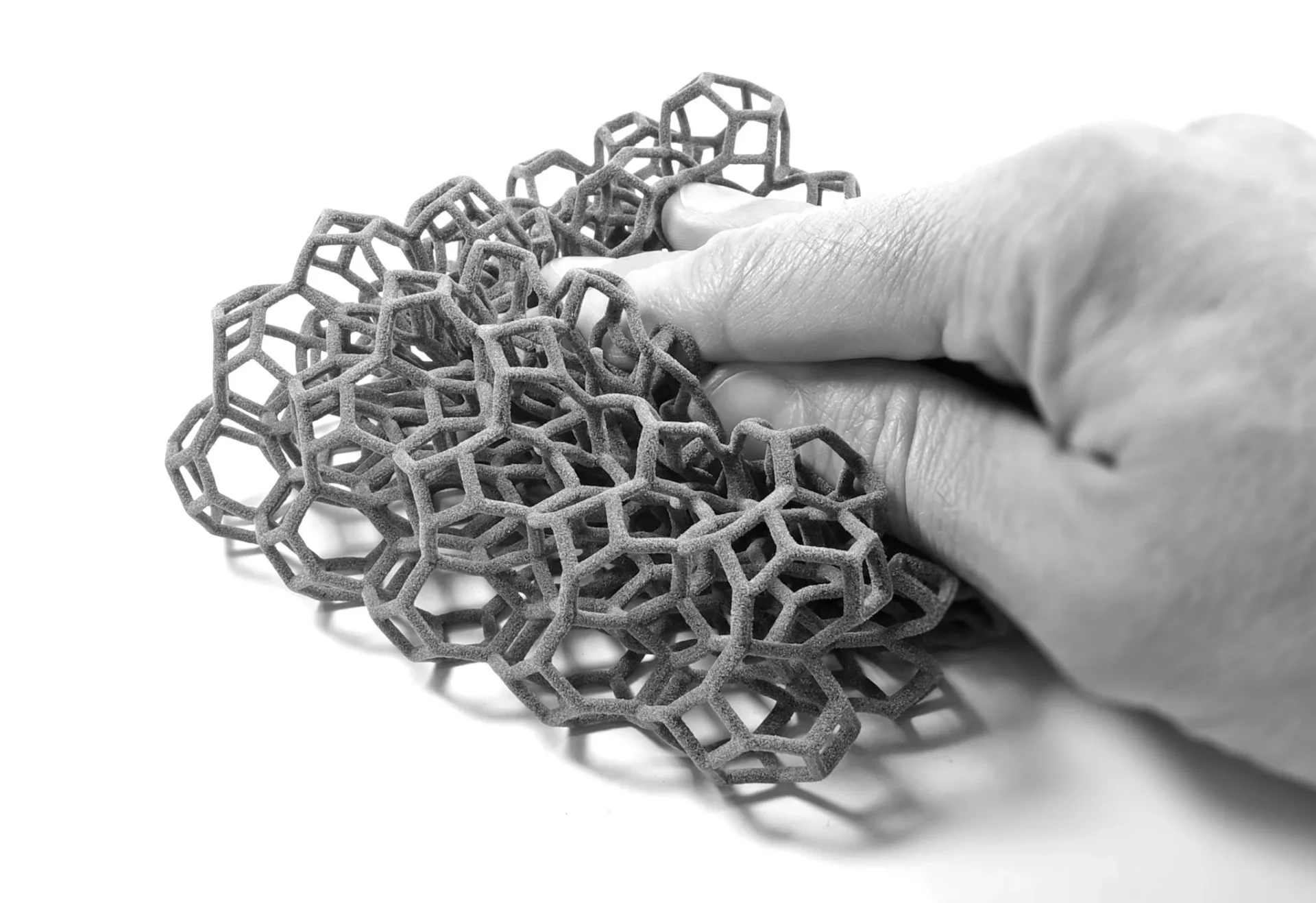
What Does Design Freedom Mean in the Context of SLS?
Design freedom refers to the ability to create complex, intricate, and highly detailed parts without the constraints typically associated with traditional manufacturing processes. In conventional manufacturing, creating complex geometries can be a challenging and costly endeavor, often requiring multiple stages of production and assembly. SLS, however, eliminates these barriers by allowing for the seamless creation of intricate designs in a single build process.
Key Elements of Design Freedom in SLS:
- Complex Geometries: SLS enables the creation of parts with highly intricate details, including internal structures, lattice designs, and overhangs.
- No Need for Support Structures: Unlike other 3D printing technologies, SLS does not require support structures, as the unsintered powder naturally supports the parts during the build process.
- Customization: SLS allows for the easy customization of parts, making it ideal for bespoke and low-volume production.
How Does SLS Allow for Complex Geometries?
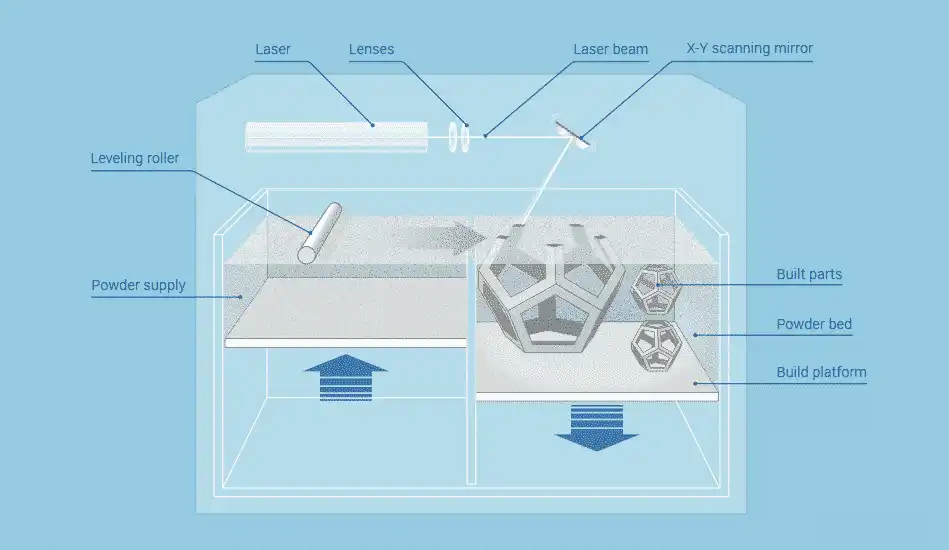
The capability to produce complex geometries is one of the standout features of SLS 3D printing. This is primarily due to the layer-by-layer nature of the process and the absence of support structures. Here’s how SLS makes complex designs possible:
- Layer-by-Layer Construction: SLS builds parts one layer at a time, allowing for precise control over each section of the design.
- Powder Bed Fusion: The unsintered powder acts as a natural support, enabling the creation of overhangs and intricate internal features without the need for additional support structures.
- High Precision Lasers: The use of high-precision lasers ensures that even the most detailed aspects of a design can be accurately reproduced.
Examples of Intricate Designs Made Possible by SLS
- Lattice Structures: Lightweight yet strong, lattice structures are often used in aerospace and automotive applications for weight reduction without compromising strength.
- Internal Channels: Complex fluid or air channels can be integrated into parts, which is particularly useful in the medical and cooling systems industries.
- Customized Medical Implants: SLS allows for the production of patient-specific implants with intricate geometries tailored to individual anatomy.
Application | Example Design | Benefits |
Aerospace | Lattice structures | Weight reduction, improved strength |
Medical | Customized implants | Personalized fit, improved patient outcomes |
Automotive | Internal cooling channels | Enhanced performance, efficiency |
Case Studies or Success Stories Showcasing Design Freedom
Case Study 1: General Electric Aviation
Project: Fuel Nozzle for Jet Engines
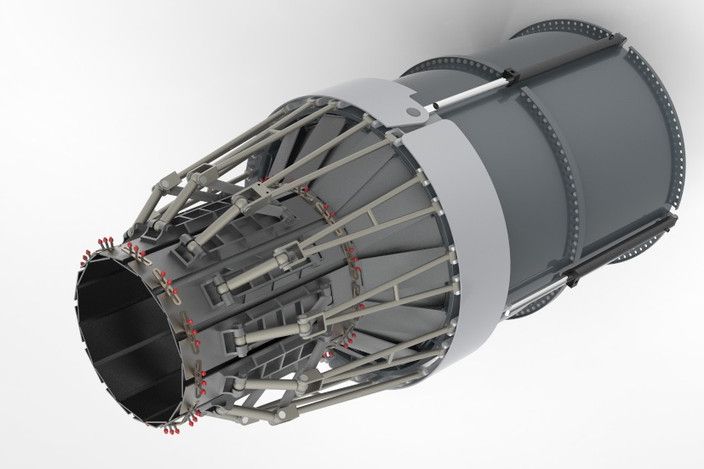
Challenge: Traditional manufacturing methods required 20 different parts to be assembled.
Solution: Using SLS, GE Aviation was able to consolidate the design into a single part with complex internal channels for fuel distribution.
Outcome: The new fuel nozzle was 25% lighter and five times more durable than its traditionally manufactured counterpart.
Case Study 2: Adidas Futurecraft 4D
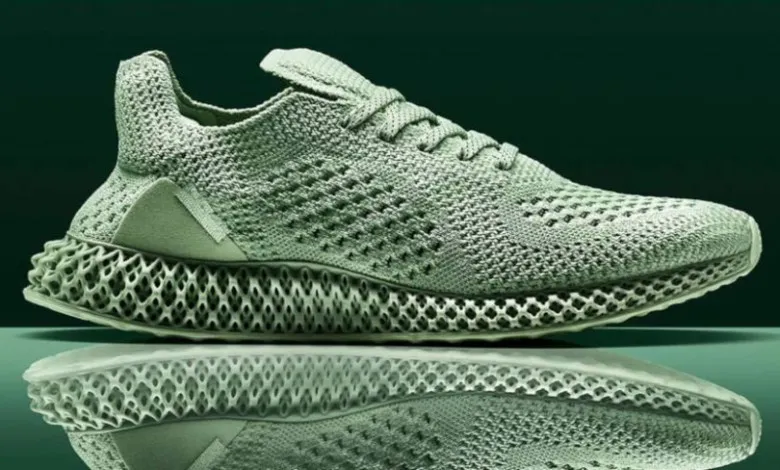
Project: Customized midsoles for athletic shoes
Challenge: Creating intricate lattice structures for optimal cushioning and performance.
Solution: SLS enabled the production of midsoles with complex lattice designs tailored to individual athletes’ needs.
Outcome: Enhanced performance and comfort, with the ability to rapidly iterate designs.
In conclusion, the design freedom offered by SLS 3D printing is a game-changer for various industries. From aerospace to medical applications, the ability to create complex, customized, and high-performance parts without the constraints of traditional manufacturing opens up new avenues for innovation and efficiency. By embracing the capabilities of SLS, designers and engineers can push the boundaries of what’s possible, creating products that were once only imaginable.
Functional Prototypes and End-Use Parts with SLS 3D Printing
Selective Laser Sintering (SLS) 3D printing has radically transformed the way we approach both prototyping and end-use part production. Its unique capabilities enable the creation of highly functional prototypes and durable, performance-ready end-use parts. But what exactly distinguishes prototypes from end-use parts, and how does SLS excel in producing both? Let’s dive into the specifics to better understand the versatility of SLS in modern manufacturing.
What is the Difference Between Prototypes and End-Use Parts?
When discussing manufacturing, it’s essential to differentiate between prototypes and end-use parts:
- Prototypes: These are early samples, models, or releases of a product built to test a concept or process. Prototyping is crucial for validating design, fit, and function before full-scale production.
- End-Use Parts: These are the final products or components ready for actual use in their intended applications. They must meet strict performance, durability, and quality standards.
How Does SLS Produce Functional Prototypes?
SLS is particularly well-suited for producing functional prototypes that closely mimic the properties of production parts. This ability allows designers to conduct rigorous testing and iterations, significantly accelerating the development cycle.
Steps to Creating Functional Prototypes with SLS:
- Design: Begin with a detailed 3D CAD model of the prototype.
- Material Selection: Choose a material that closely matches the intended end-use material properties.
- Sintering Process: Use SLS to build the prototype layer by layer, ensuring high precision and detail.
- Post-Processing: Finish the prototype with necessary post-processing steps such as cleaning, surface finishing, and painting if required.
Benefits of Functional SLS Prototypes:
- High Fidelity: SLS prototypes closely replicate the final product’s mechanical and physical properties, enabling accurate testing.
- Speed: Rapid iteration is possible due to the quick turnaround times of SLS printing.
- Cost-Efficiency: Reduces the need for expensive molds and tooling typically required in traditional prototyping methods.
Examples of End-Use Parts Manufactured with SLS
SLS technology isn’t limited to prototyping; it’s also highly effective for producing end-use parts. These parts can range from high-performance automotive components to customized consumer products.
Notable Examples:
- Automotive Components: Heat-resistant parts like air ducts, intake manifolds, and brackets.
- Consumer Electronics: Durable and precise components for gadgets and devices.
- Medical Devices: Custom prosthetics, implants, and surgical guides tailored to patient-specific needs.
- Fashion and Accessories: Bespoke jewelry, eyewear, and fashion items with intricate details.
Industry | Example End-Use Parts | Key Benefits |
Automotive | Air ducts, brackets, manifolds | Heat resistance, durability |
Consumer Electronics | Cases, internal components | Precision, durability |
Medical | Custom prosthetics, implants | Personalization, biocompatibility |
Fashion | Jewelry, eyewear, accessories | Customization, intricate design |
Discussion on the Durability and Performance of SLS Parts
The durability and performance of SLS-produced parts are among the technology’s most significant strengths. The sintering process creates parts with excellent mechanical properties, making them suitable for both low-volume production and high-stress applications.
Key Advantages:
- Strength: SLS parts exhibit high tensile strength and impact resistance, often comparable to injection-molded parts.
- Heat Resistance: Materials like glass-filled nylon provide exceptional thermal stability, making them suitable for high-temperature environments.
- Flexibility: Thermoplastic polyurethane (TPU) parts offer rubber-like flexibility and resilience, ideal for components that require elasticity.
Real-World Applications:
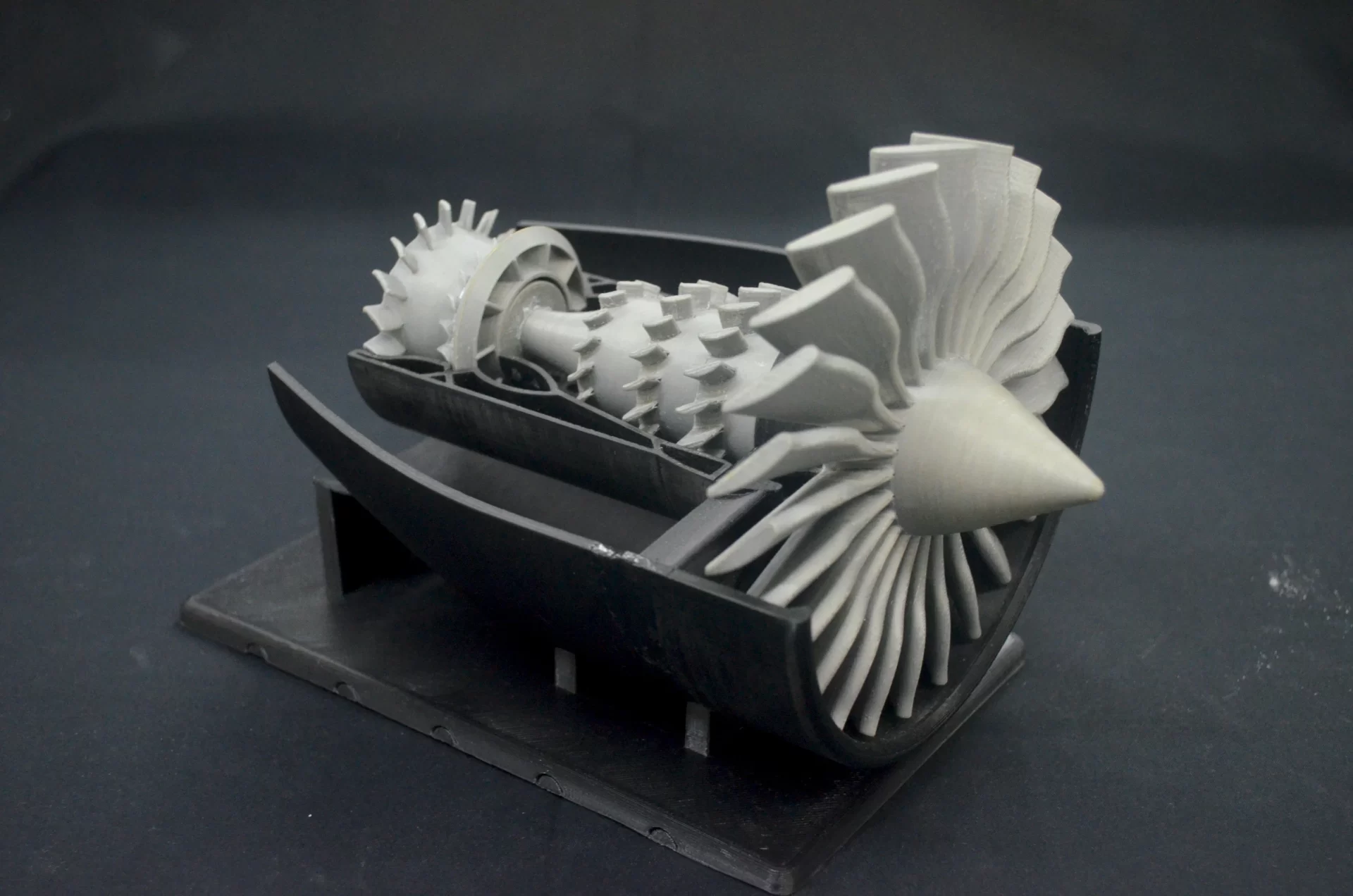
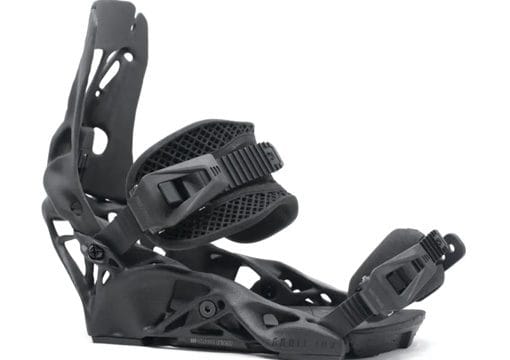
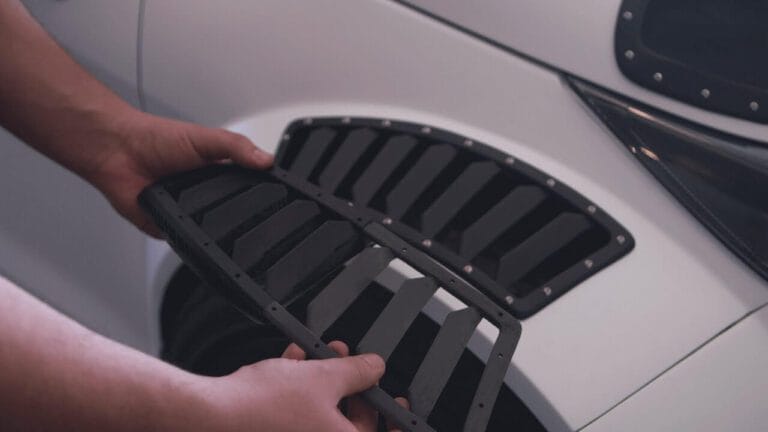
- Aerospace: Lightweight yet strong parts such as ducting systems and brackets that can withstand extreme conditions.
- Medical: Personalized implants and surgical guides that need to maintain integrity and performance during medical procedures.
- Automotive: Durable components that endure the stresses of automotive environments, such as engine bay parts.
In conclusion, SLS 3D printing is a versatile and powerful tool capable of producing both functional prototypes and robust end-use parts. By leveraging the unique advantages of SLS, manufacturers can optimize their development cycles, reduce costs, and produce high-quality, performance-ready components. Whether you’re refining a design or moving into full-scale production, SLS offers the reliability and flexibility needed to meet the demands of modern manufacturing. Embracing SLS technology opens up new possibilities for innovation, efficiency, and excellence in product development.
Post-Processing Techniques in SLS 3D Printing
Selective Laser Sintering (SLS) 3D printing is renowned for its ability to produce complex, high-quality parts. However, the journey from the print bed to a finished product often involves several post-processing steps. These techniques not only enhance the aesthetic appeal of the parts but also improve their mechanical properties, making them suitable for a wide range of applications. In this section, we will explore the various post-processing techniques used in SLS 3D printing, their benefits, and how they contribute to the overall quality of the final product.
Why is Post-Processing Important in SLS?
Post-processing is essential for several reasons:
- Surface Finish: SLS-printed parts often have a rough surface finish due to the powder-based nature of the process. Post-processing techniques can significantly smoothen the surface.
- Mechanical Properties: Techniques like annealing can enhance the mechanical properties of SLS parts, making them more durable and suitable for end-use applications.
- Aesthetic Appeal: Post-processing can improve the visual appearance of the parts, making them more appealing for consumer products.
- Functional Enhancements: Techniques like coating can add functional properties such as increased wear resistance or electrical conductivity.
Common Post-Processing Techniques for SLS
1. Depowdering
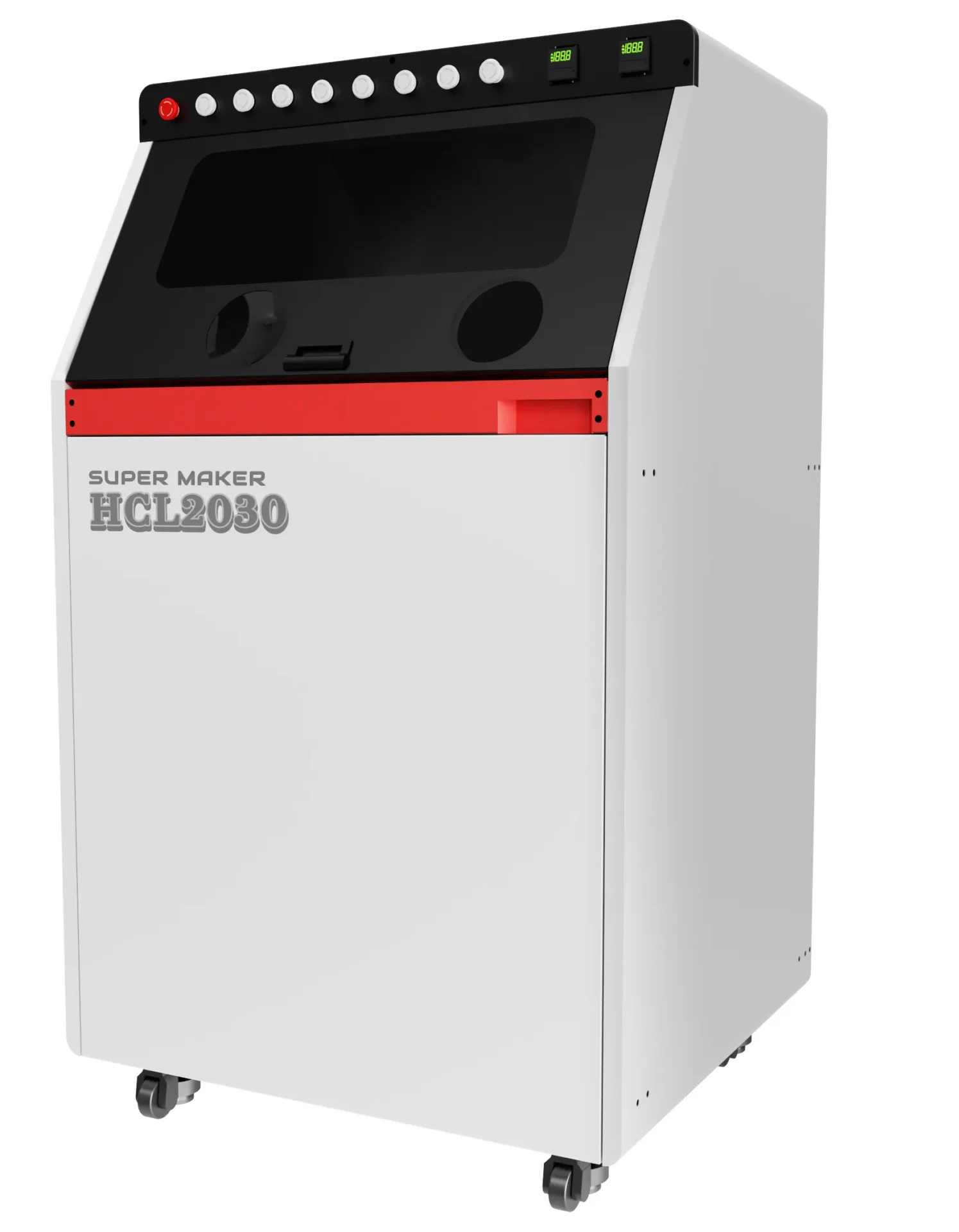
Depowdering is the first and most critical step in the post-processing of SLS parts. It involves the removal of excess powder that surrounds and supports the printed part during the build process.
- Methods:
- Manual Brushing: Using brushes to remove loose powder.
- Compressed Air Blowing: Blowing away powder with compressed air.
- Vacuuming: Using a vacuum to suck away excess powder.
- Benefits:
- Prepares the part for further post-processing steps.
- Reduces the risk of powder contamination during subsequent processes.
2. Surface Smoothing
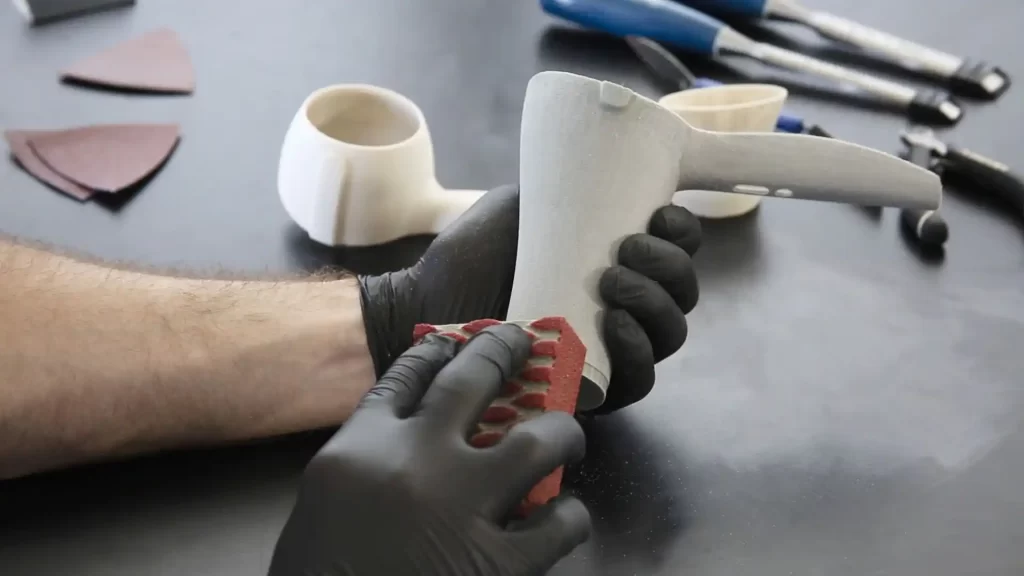
Surface smoothing aims to improve the surface finish of SLS parts by reducing the rough texture.
- Methods:
- Media Blasting: Using abrasive media to smoothen the surface.
- Tumbling: Placing the parts in a vibrating machine filled with abrasive media.
- Chemical Smoothing: Using chemicals to dissolve surface imperfections.
- Benefits:
- Achieves a smoother surface finish.
- Enhances the aesthetic appeal of the part.
3. Dyeing and Painting
Dyeing and painting are used to add color to SLS parts, making them suitable for consumer products and promotional items.
- Methods:
- Dyeing: Submerging the part in a dye solution.
- Painting: Applying paint using sprays or brushes.
- Benefits:
- Customizable appearance.
- Improved aesthetics for consumer-facing products.
4. Infiltration and Coating
Infiltration and coating add functional properties to SLS parts, such as increased strength or resistance to environmental factors.
- Methods:
- Epoxy Infiltration: Filling the porous surface with epoxy to increase strength.
- Ceramic Coating: Applying a ceramic layer for heat resistance.
- Metal Plating: Adding a metal layer for enhanced durability and conductivity.
- Benefits:
- Improved mechanical properties.
- Added functional properties like heat resistance or electrical conductivity.
5. Annealing
Annealing is a heat treatment process that enhances the mechanical properties of SLS parts.
- Methods:
- Controlled Heating: Heating the part to a specific temperature and then cooling it slowly.
- Benefits:
- Increases tensile strength and impact resistance.
- Reduces internal stresses within the part.
Post-Processing Technique | Methods | Key Benefits |
Depowdering | Manual brushing, compressed air, vacuuming | Prepares part for further steps |
Surface Smoothing | Media blasting, tumbling, chemical smoothing | Improved surface finish |
Dyeing and Painting | Dyeing, painting | Customizable appearance |
Infiltration and Coating | Epoxy infiltration, ceramic coating, metal plating | Enhanced mechanical properties |
Annealing | Controlled heating | Increased strength, reduced stress |
Case Study: Post-Processing in Action
Case Study: Custom Eyewear Production
Project: Production of custom eyewear frames.
Challenge: Achieving a smooth, aesthetically pleasing surface finish while maintaining durability.
Solution:
- Depowdering: Manual brushing to remove excess powder.
- Surface Smoothing: Tumbling to achieve a smooth surface finish.
- Painting: Applying a custom color paint for a premium look.
- Infiltration: Epoxy infiltration to enhance durability.
Outcome: The final product had a high-quality finish, improved durability, and a customized appearance that met consumer expectations.
So, post-processing techniques are a vital part of the SLS 3D printing workflow, enhancing the quality, functionality, and aesthetic appeal of the final parts. By understanding and implementing these techniques, manufacturers can produce high-performance, visually appealing products that meet industry standards and consumer expectations. Embracing these post-processing methods ensures that SLS-printed parts are not only ready for functional testing but also fit for end-use applications, opening up new possibilities for innovation and excellence in various industries.
Cost Efficiency and Time Savings with SLS 3D Printing
Selective Laser Sintering (SLS) 3D printing stands out not only for its design flexibility and material versatility but also for its cost efficiency and time-saving capabilities. These factors are critical in today’s fast-paced market, where companies seek to bring high-quality products to market quickly and economically. In this section, we will delve into how SLS 3D printing offers substantial cost savings and time efficiency compared to traditional manufacturing methods.
How SLS 3D Printing Reduces Costs
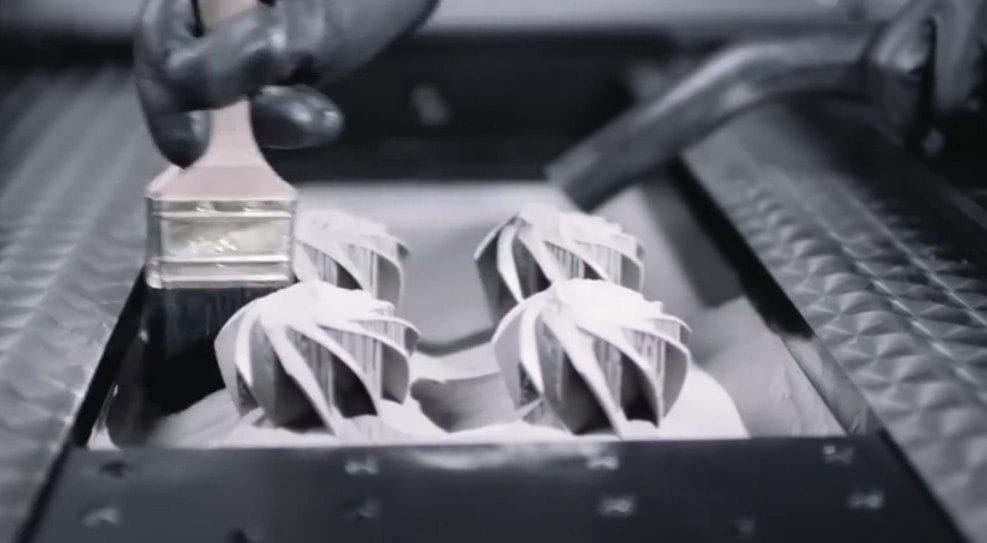
SLS 3D printing reduces costs in several key areas:
1. Elimination of Tooling Costs
Traditional manufacturing often necessitates expensive tooling, such as molds and dies, which can be particularly costly for low-volume production. SLS eliminates the need for tooling, enabling cost-effective manufacturing even for small batches.
- Benefits:
- No Initial Tooling Investment: Significant cost savings, especially for prototypes or small production runs.
- Flexibility in Design Changes: Easy and low-cost modifications to designs without the need to alter tooling.
2. Reduction in Material Waste
Traditional subtractive manufacturing methods often result in significant material waste. SLS, being an additive process, uses only the material necessary to build the part, with excess powder remaining recyclable for future builds.
- Benefits:
- Efficient Material Use: Minimizes waste and reduces material costs.
- Sustainability: Promotes environmentally friendly practices by reducing waste.
3. Lower Labor Costs
SLS 3D printing automates much of the manufacturing process, reducing the need for manual intervention and, consequently, labor costs.
- Benefits:
- Automation: Reduces reliance on manual labor, lowering overall production costs.
- Efficiency: Frees up human resources to focus on other value-added tasks.
4. Consolidation of Parts
SLS allows for the integration of complex geometries and multiple components into a single part, reducing the need for assembly and the associated costs.
- Benefits:
- Reduced Assembly Time: Fewer parts to assemble means less labor and lower costs.
- Improved Reliability: Fewer joints and connections reduce the risk of part failure.
Cost Factor | Traditional Manufacturing | SLS 3D Printing | Key Savings |
Tooling Costs | High | None | Significant savings, especially for low-volume production |
Material Waste | High | Minimal | Reduced material costs |
Labor Costs | High | Low | Lower overall production costs |
Part Consolidation | Low | High | Reduced assembly costs and improved reliability |
How SLS 3D Printing Saves Time
Speed to market is a crucial factor for competitive advantage. SLS 3D printing accelerates the manufacturing process in several ways:
1. Rapid Prototyping
SLS enables quick iteration of design prototypes, allowing for rapid validation and refinement of concepts.
- Benefits:
- Fast Turnaround: Prototypes can be produced in a matter of hours or days, rather than weeks.
- Quick Iterations: Rapid testing and adjustments speed up the development cycle.
2. Short Lead Times
The absence of tooling and the ability to produce parts directly from digital files significantly reduce lead times.
- Benefits:
- Direct Manufacturing: Parts can be produced on-demand, reducing the waiting time associated with tooling and setup.
- Flexibility: Easy adjustments to designs without delays.
3. On-Demand Production
SLS allows for the production of parts as needed, eliminating the need for large inventories and the associated storage costs.
- Benefits:
- Inventory Management: Reduces the need for warehousing and inventory holding costs.
- Just-in-Time Production: Produces parts exactly when they are needed, enhancing operational efficiency.
4. Streamlined Post-Processing
Advancements in post-processing techniques have made it quicker and easier to finish SLS parts, further reducing the overall production time.
- Benefits:
- Efficient Finishing: Automated and efficient post-processing methods speed up the final stages of production.
- Consistency: Ensures high-quality finishes in less time.
Time Factor | Traditional Manufacturing | SLS 3D Printing | Key Time Savings |
Prototyping Speed | Slow | Fast | Rapid iteration and validation |
Lead Times | Long | Short | Reduced setup and tooling times |
Production Flexibility | Limited | High | On-demand production and quick adjustments |
Post-Processing | Time-Consuming | Streamlined | Efficient finishing processes |
Case Study: Time and Cost Savings in Action
Case Study: Aerospace Component Manufacturing
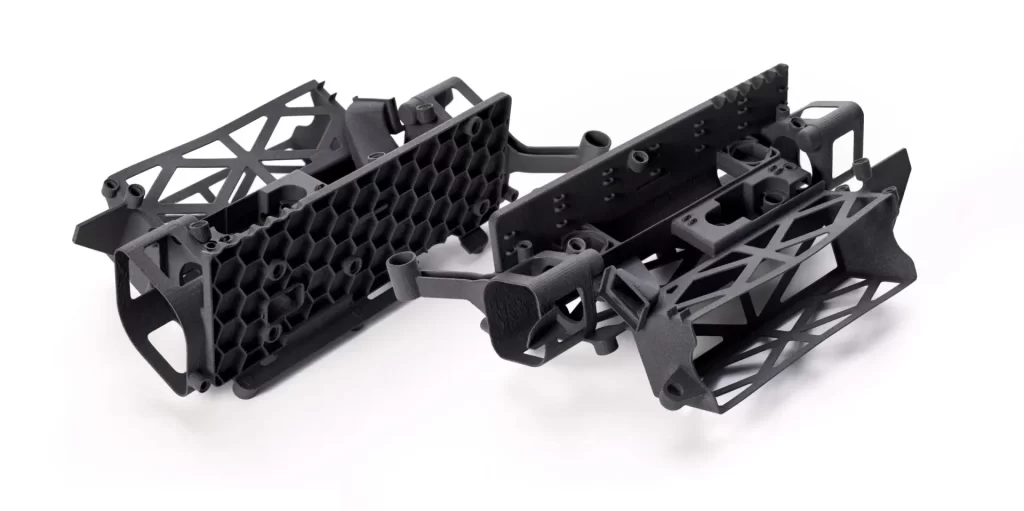
Project: Production of complex ducting systems for aerospace applications.
Challenge: Traditional methods required multiple parts and extensive assembly, leading to high costs and long lead times.
Solution:
- Tooling Elimination: SLS allowed for the direct production of parts without expensive tooling.
- Material Efficiency: Reduced material waste by using only the necessary amount of powder.
- Rapid Prototyping: Quickly iterated designs to optimize performance.
- Part Consolidation: Combined multiple components into a single, complex part, reducing assembly time.
Outcome: The project saw a 40% reduction in production costs and a 60% decrease in lead times, enabling faster delivery and significant cost savings.
SLS 3D printing offers unparalleled cost efficiency and time savings, making it an attractive option for modern manufacturing. By eliminating the need for tooling, reducing material waste, lowering labor costs, and enabling rapid prototyping and on-demand production, SLS empowers companies to bring high-quality products to market faster and more economically. Embracing the advantages of SLS not only enhances operational efficiency but also provides a competitive edge in a rapidly evolving market landscape.
Quality Control and Assurance in SLS 3D Printing
Ensuring the highest quality of 3D-printed parts is critical, especially in industries where reliability and performance are paramount. Selective Laser Sintering (SLS) 3D printing, with its ability to produce highly complex and functional parts, requires rigorous quality control and assurance measures to meet stringent standards. In this section, we will explore the various techniques and practices used to maintain quality in SLS 3D printing, from pre-print preparations to post-production inspections.
Importance of Quality Control in SLS
Quality control in SLS 3D printing is essential for several reasons:
- Consistency: Ensures that each printed part meets the required specifications and standards.
- Reliability: Guarantees the durability and performance of the parts, which is crucial for critical applications.
- Compliance: Ensures that parts meet industry-specific regulatory requirements and standards.
- Customer Satisfaction: Delivers high-quality products that meet or exceed customer expectations.
Pre-Print Quality Control Measures
1. Material Quality
The quality of the raw material (powder) used in SLS printing is a fundamental factor influencing the final part’s quality.
- Monitoring: Regular checks and monitoring of powder properties such as particle size, shape, and distribution.
- Storage: Proper storage conditions to maintain material quality and prevent contamination or degradation.
2. Design Validation
Before printing, the design must be validated to ensure it is suitable for the SLS process.
- Simulation: Use of simulation software to predict how the part will behave during printing and identify potential issues.
- Design for Manufacturability (DFM): Ensuring the design is optimized for the SLS process, including considerations for support structures and part orientation.
3. Machine Calibration
Regular calibration of the SLS printer ensures consistent performance and accuracy.
- Laser Calibration: Ensuring the laser is properly calibrated to maintain correct energy output and focus.
- Bed Leveling: Checking and adjusting the build bed to ensure it is level, which is critical for uniform layer deposition.
Pre-Print Measure | Key Actions | Benefits |
Material Quality | Monitoring properties, proper storage | High-quality material consistency |
Design Validation | Simulation, DFM | Optimized designs, reduced errors |
Machine Calibration | Laser calibration, bed leveling | Consistent printing accuracy |
In-Print Quality Control Techniques
1. Real-Time Monitoring
Real-time monitoring of the printing process helps detect and address issues as they arise.
- Cameras and Sensors: Use of high-resolution cameras and sensors to monitor the build process.
- Layer Inspection: Automated layer-by-layer inspection to identify defects or anomalies during printing.
2. Environmental Control
Maintaining a controlled environment within the build chamber is crucial for consistent quality.
- Temperature and Humidity Control: Ensuring the build chamber maintains optimal temperature and humidity levels.
- Airflow Management: Proper airflow to prevent contamination and ensure even cooling.
In-Print Technique | Key Actions | Benefits |
Real-Time Monitoring | Cameras, sensors, layer inspection | Immediate issue detection and correction |
Environmental Control | Temperature, humidity, airflow management | Consistent build quality |
Post-Print Quality Assurance Methods
1. Depowdering and Cleaning
Effective removal of excess powder is crucial for part quality.
- Manual and Automated Methods: Use of brushes, air blowers, or automated depowdering systems.
- Thorough Cleaning: Ensuring all residual powder is removed to prevent defects in the final part.
2. Dimensional Inspection
Measuring the dimensions of printed parts to ensure they meet design specifications.
- Coordinate Measuring Machines (CMM): High-precision machines that measure the dimensions of the parts.
- Laser Scanners: Non-contact measurement systems for detailed, accurate dimensional analysis.
3. Mechanical Testing
Testing the mechanical properties of printed parts to ensure they meet required standards.
- Tensile and Compression Testing: Evaluating the part’s strength and elasticity.
- Impact Testing: Assessing the part’s ability to withstand sudden forces or impacts.
4. Non-Destructive Testing (NDT)
Techniques that inspect the integrity of the part without causing damage.
- Ultrasonic Testing: Using sound waves to detect internal defects.
- X-ray Inspection: Identifying internal inconsistencies or voids.
Post-Print Method | Key Actions | Benefits |
Depowdering and Cleaning | Manual/automated methods, thorough cleaning | Removes residual powder, prevents defects |
Dimensional Inspection | CMM, laser scanners | Ensures parts meet design specifications |
Mechanical Testing | Tensile, compression, impact testing | Verifies mechanical properties |
Non-Destructive Testing | Ultrasonic, X-ray inspection | Detects internal defects without damage |
Case Study: Quality Assurance in Medical Device Production
Case Study: Custom Orthopedic Implants
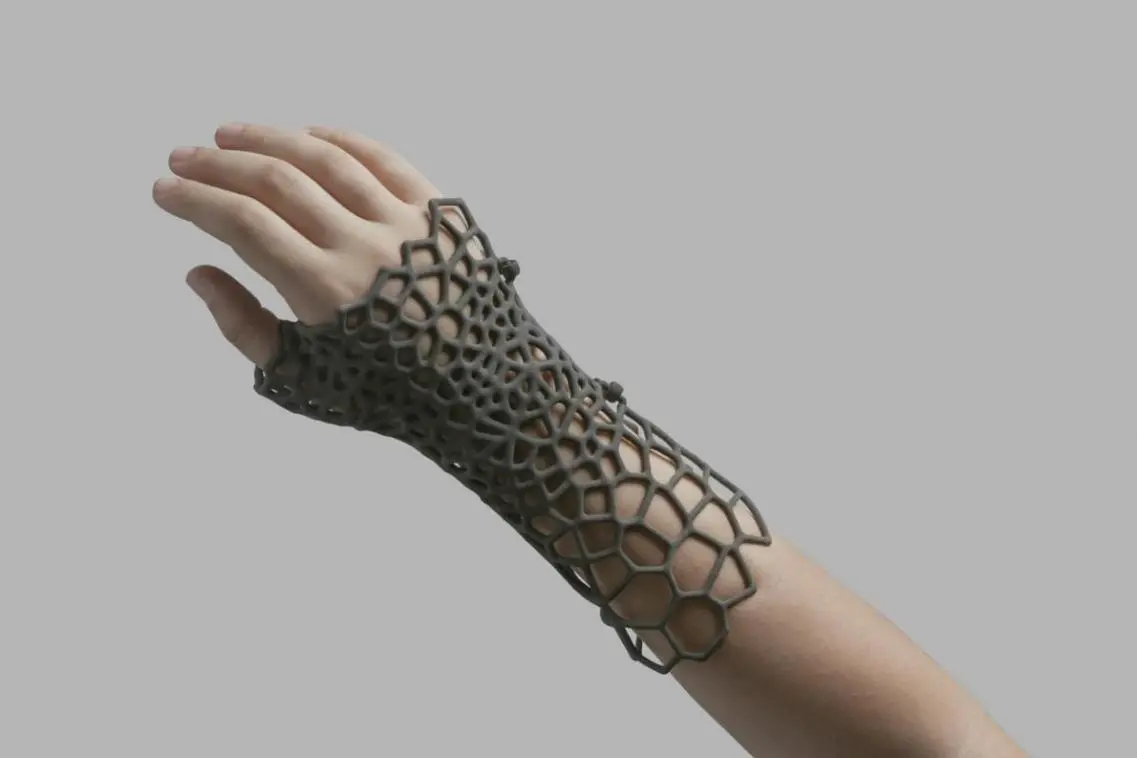
Project: Production of custom orthopedic implants.
Challenge: Ensuring that each implant meets stringent medical standards and is free from defects.
Solution:
- Material Quality: Strict monitoring of powder properties and maintaining optimal storage conditions.
- Design Validation: Extensive simulation and DFM analysis to ensure the design is suitable for SLS printing.
- Machine Calibration: Regular calibration of the printer to maintain accuracy.
- Real-Time Monitoring: Use of cameras and sensors to monitor the build process in real time.
- Post-Print Inspection: Thorough depowdering, dimensional inspection using CMM, and mechanical testing to ensure each implant meets required standards.
Outcome: The production process yielded high-quality, reliable implants that met all regulatory requirements, ensuring patient safety and satisfaction.
Thus, quality control and assurance in SLS 3D printing are indispensable for producing reliable, high-performance parts. By implementing rigorous pre-print, in-print, and post-print quality measures, manufacturers can ensure consistency, reliability, and compliance with industry standards. These practices not only enhance the overall quality of SLS-printed parts but also build customer trust and satisfaction, paving the way for broader adoption of SLS technology in various critical applications. Embracing comprehensive quality control methods ensures that SLS 3D printing continues to be a reliable and effective manufacturing solution in the ever-evolving landscape of modern industry.
Sustainable Practices in SLS 3D Printing
Selective Laser Sintering (SLS) 3D printing is not only revolutionizing manufacturing with its flexibility and efficiency but also paving the way for more sustainable production practices. As industries become increasingly aware of their environmental impact, SLS offers several key advantages that contribute to sustainability. In this section, we will explore how SLS 3D printing promotes sustainable practices, from material usage to energy efficiency, and examine its role in creating a more eco-friendly manufacturing landscape.
Material Efficiency and Recycling
1. Reduced Material Waste
One of the primary benefits of SLS 3D printing is its ability to minimize material waste. Unlike traditional subtractive manufacturing methods, which often result in significant waste, SLS uses only the material necessary to build the part.
- Additive Process: SLS builds parts layer by layer, using only the required amount of material.
- Surplus Powder Reuse: Unfused powder can be collected, sieved, and reused in future print jobs, reducing raw material consumption.
2. Recyclable Materials
Many of the materials used in SLS printing are recyclable, further enhancing sustainability.
- Nylon Powders: Commonly used in SLS printing, nylon powders can be recycled and reused, reducing environmental impact.
- Biodegradable Options: Some SLS materials are biodegradable, offering additional eco-friendly options for manufacturers.
Material Practice | Key Actions | Benefits |
Reduced Material Waste | Additive process, surplus powder reuse | Minimizes waste, reduces raw material use |
Recyclable Materials | Use of recyclable and biodegradable materials | Decreases environmental impact |
Energy Efficiency
1. Efficient Manufacturing Process
SLS 3D printing is inherently energy-efficient, particularly when compared to many traditional manufacturing methods.
- Consolidated Production: SLS can produce complex parts in a single build, reducing the need for multiple manufacturing steps and the associated energy consumption.
- On-Demand Production: Producing parts as needed reduces energy spent on maintaining large inventories and prevents overproduction.
2. Optimized Machine Use
Advanced SLS machines are designed to optimize energy use, further enhancing their sustainability.
- Energy-Efficient Lasers: Modern SLS machines use lasers that are designed to be energy-efficient while maintaining high performance.
- Smart Scheduling: Optimizes print jobs to maximize machine use and minimize downtime, improving overall energy efficiency.
Energy Practice | Key Actions | Benefits |
Efficient Manufacturing | Consolidated production, on-demand production | Reduces energy consumption |
Optimized Machine Use | Energy-efficient lasers, smart scheduling | Enhances overall energy efficiency |
Design Innovation for Sustainability
1. Lightweight Structures
SLS allows for the creation of complex, lightweight structures that use less material without compromising strength or functionality.
- Topology Optimization: Software tools can optimize part designs to minimize material use while maintaining structural integrity.
- Complex Geometries: SLS can produce intricate designs that traditional methods can’t, reducing material usage and weight.
2. Part Consolidation
By integrating multiple components into a single part, SLS reduces the need for assembly and minimizes the overall material and energy footprint.
- Reduced Assembly: Fewer parts mean reduced assembly requirements and less energy consumption.
- Streamlined Supply Chains: Simplified part designs can streamline logistics and reduce transportation-related emissions.
Design Practice | Key Actions | Benefits |
Lightweight Structures | Topology optimization, complex geometries | Minimizes material use, reduces weight |
Part Consolidation | Reduced assembly, streamlined supply chains | Lowers energy and material footprint |
Case Study: Sustainable Manufacturing in Consumer Products
Case Study: Eco-Friendly Sports Equipment
Project: Development of sustainable sports equipment using SLS 3D printing.
Challenge: Reduce environmental impact while maintaining performance and durability.
Solution:
- Material Efficiency: Used recyclable nylon powders, promoting a circular material lifecycle.
- Energy Efficiency: Optimized machine use and employed energy-efficient lasers for production.
- Lightweight Design: Implemented topology optimization to create lightweight yet strong equipment.
- Part Consolidation: Designed equipment with fewer components to streamline production and reduce waste.
Outcome: The project successfully delivered high-performance, eco-friendly sports equipment, demonstrating a significant reduction in environmental impact and setting a benchmark for sustainable manufacturing practices in the industry.
SLS 3D printing is at the forefront of sustainable manufacturing practices, offering significant advantages in material efficiency, energy consumption, and design innovation. By minimizing waste, optimizing energy use, and enabling more sustainable product designs, SLS is helping industries align with environmental goals and reduce their ecological footprint. As sustainability becomes a crucial factor in manufacturing, embracing SLS 3D printing can lead to both economic and environmental benefits, driving the industry towards a greener future.
Innovations and Future Trends in SLS 3D Printing
Selective Laser Sintering (SLS) 3D printing continues to be at the cutting edge of manufacturing technology, with ongoing innovations and emerging trends that promise to expand its capabilities and applications. As industries push the limits of what can be achieved with 3D printing, SLS is set to play a pivotal role in driving future advancements. In this section, we will explore the latest innovations in SLS technology and examine the trends that are shaping its future.
Advanced Materials
1. High-Performance Polymers
The development of new high-performance polymers for SLS printing is expanding the range of applications and enhancing the mechanical properties of printed parts.
- Engineering-Grade Materials: New polymers offer enhanced strength, heat resistance, and chemical stability.
- Biocompatible Options: Materials that are safe for medical and dental applications are becoming more prevalent.
2. Metal and Composite SLS
Innovations in material science are enabling the use of metals and composite materials in SLS printing, offering new possibilities for industrial applications.
- Metallic SLS: Development of metal-infused powders for high-strength and heat-resistant parts.
- Composite Materials: Integration of fibers and other reinforcements into powders to enhance performance properties.
Material Innovation | Key Developments | Benefits |
High-Performance Polymers | Engineering-grade, biocompatible materials | Wider application range, improved properties |
Metal and Composite SLS | Metallic and composite powders | Enhanced strength, new industrial uses |
Technological Enhancements
1. Improved Precision and Resolution
Advancements in laser technology and scanning systems are leading to higher precision and better resolution in SLS 3D printing.
- Fine Laser Control: Improved laser diodes offer precise control for detailed printing.
- Advanced Scanning Algorithms: Enhanced algorithms result in more accurate layer formation and smoother surfaces.
2. Faster Print Speeds
The development of faster printing technologies is reducing production times and increasing throughput.
- High-Speed Lasers: Faster lasers can sinter material more quickly without sacrificing quality.
- Multi-Laser Systems: Use of multiple lasers simultaneously to speed up the printing process.
Technological Enhancement | Key Developments | Benefits |
Improved Precision and Resolution | Fine laser control, advanced algorithms | Higher quality parts, detailed features |
Faster Print Speeds | High-speed and multi-laser systems | Reduced production times, increased throughput |
Automation and Integration
1. End-to-End Automation
Integration of automation in the entire SLS workflow is streamlining production and improving efficiency.
- Automated Post-Processing: Systems that automate depowdering and finishing processes.
- Integrated Software Solutions: Software that manages the entire print cycle, from design to the final product.
2. Industry 4.0 and IoT
The implementation of Industry 4.0 principles and IoT connectivity in SLS machines enhances real-time monitoring and data-driven decision-making.
- Smart Manufacturing: Real-time adjustments and monitoring for optimal performance.
- Predictive Maintenance: IoT sensors help predict and prevent machine failures.
Automation and Integration | Key Developments | Benefits |
End-to-End Automation | Automated processes, integrated software | Streamlined production, reduced labor costs |
Industry 4.0 and IoT | Smart manufacturing, predictive maintenance | Enhanced efficiency, reduced downtime |
Emerging Applications
1. Mass Customization
SLS technology is enabling mass customization, particularly in industries like automotive, consumer goods, and healthcare.
- Personalized Products: Ability to produce unique, custom-tailored products in large volumes.
- Rapid Prototyping and Production: Accelerating product development cycles with on-demand manufacturing.
2. Sustainability and Circular Economy
SLS is playing a significant role in promoting sustainability and the circular economy by enabling more sustainable materials and efficient production processes.
- Eco-Friendly Materials: Development of sustainable material options for SLS.
- Resource Efficiency: Efficient use of materials and energy in production processes.
Emerging Application | Key Developments | Benefits |
Mass Customization | Personalized products, rapid prototyping | Custom solutions, faster market entry |
Sustainability and Circular Economy | Eco-friendly materials, resource efficiency | Reduced environmental impact |
Case Study: Futuristic Application in Aerospace
Case Study: Advanced Aircraft Components
Project: Development of next-generation aircraft components using SLS 3D printing.
Challenge: Achieving high performance and lightweight structures while reducing environmental impact.
Solution:
- High-Performance Materials: Utilization of advanced polymers and metal composites to withstand extreme conditions.
- Precision Printing: Implementation of high-resolution and fast print technologies for complex geometries.
- Integrated Systems: Use of automated and IoT-enabled systems for efficient production and real-time monitoring.
- Sustainable Practices: Adoption of eco-friendly materials and energy-efficient methods.
Outcome: Successfully produced components that exceeded performance expectations, reduced weight, and minimized environmental impact, showcasing the future potential of SLS in aerospace innovation.
In conclusion, the future of SLS 3D printing is bright with continuous innovations and emerging trends that are reshaping the manufacturing landscape. Advances in materials, technology, and automation, along with the expansion into new applications, highlight the dynamic and evolving nature of SLS. As these innovations continue to unfold, SLS 3D printing is poised to play a pivotal role in driving industry transformation, promoting sustainability, and meeting the ever-changing demands of modern manufacturing. By harnessing the full potential of SLS, industries can unlock new opportunities and push the boundaries of what is possible in the world of 3D printing.